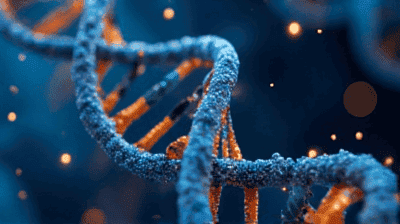
The field of genetics has seen monumental advances in recent years, with one of the most significant breakthroughs being the development of CRISPR gene editing technology. CRISPR, which stands for Clustered Regularly Interspaced Short Palindromic Repeats, has revolutionized our understanding of genetics and opened new avenues for treating diseases, from genetic disorders to certain types of cancer.
The Basics of CRISPR
What is CRISPR?
CRISPR is a natural immune system found in bacteria that helps them defend against viruses. Scientists have harnessed this system to create a powerful tool for gene editing. The CRISPR system consists of two main components:
- Guide RNA: A synthetic RNA sequence that is designed to match a specific DNA target in the genome.
- Cas9 Protein: An enzyme that acts as molecular scissors, cutting the DNA at the target location specified by the guide RNA.
When CRISPR is introduced into a cell, the guide RNA directs the Cas9 enzyme to the appropriate spot in the DNA sequence, where it creates a double-strand break. The cell's natural repair processes kick in to fix this break, allowing scientists to either disable a gene or insert a new piece of DNA.
The Discovery of CRISPR
The history of CRISPR dates back to the late 1980s when researchers first identified these unique DNA sequences in the genomes of bacteria. Further studies revealed their role in bacterial immunity, leading scientists to investigate potential applications in gene editing. In 2012, Jennifer Doudna and Emmanuelle Charpentier published a groundbreaking paper detailing how CRISPR could be used as a precise genetic engineering tool. This breakthrough has since sparked a wave of research and development across various fields.
How CRISPR Works
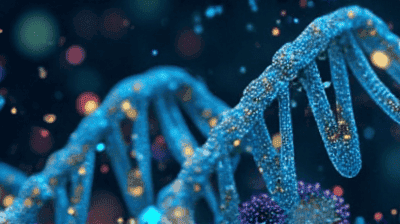
Targeting Specific Genes
One of the most remarkable features of CRISPR is its ability to target specific genes. The process begins with designing a guide RNA that corresponds to a sequence in the target gene. Once introduced into the cell, the guide RNA binds to the target DNA, leading the Cas9 enzyme to that exact location. The precision of this targeting allows for highly efficient gene editing.
Creating Double-Strand Breaks
The Cas9 enzyme, once directed to the target location by the guide RNA, induces a double-strand break in the DNA. This break is crucial because it triggers the cell's repair mechanisms to kick in. There are two primary pathways that the cell can use to repair double-strand breaks:
Non-Homologous End Joining (NHEJ): This pathway repairs breaks by directly joining the two ends of the DNA together. However, this process is error-prone and can lead to insertions or deletions, effectively knocking out the target gene.
Homologous Recombination (HR): In this pathway, a repair template (a piece of DNA with the desired sequence) can be introduced alongside the CRISPR components. The cell can use this template to accurately repair the break and incorporate new genetic material.
Applications of CRISPR in Medicine
The versatility of CRISPR technology opens the door to numerous applications in medicine. Here are some of the most promising uses:
1. Treating Genetic Disorders
CRISPR has the potential to cure genetic disorders by directly correcting mutations in a patient's DNA. Conditions such as cystic fibrosis, sickle cell disease, and muscular dystrophy are caused by specific genetic mutations, making them ideal candidates for CRISPR-based therapies. Researchers have already demonstrated the successful use of CRISPR to correct mutations in cell lines and animal models, paving the way for potential human applications.
For example, in a landmark study, researchers used CRISPR to edit the genes of patients with sickle cell disease and beta-thalassemia. By modifying a specific gene involved in hemoglobin production, they aimed to restore normal blood function. Early results showed promise, with patients experiencing significant improvements in symptoms.
2. Cancer Immunotherapy
CRISPR is also being explored as a tool for enhancing cancer immunotherapy. By editing the genes of immune cells, scientists can improve their ability to recognize and attack cancer cells. A notable example is the use of CRISPR to modify T cells, a type of immune cell, to better target and eliminate tumors.
Preclinical studies have shown that CRISPR-engineered T cells can effectively target cancers such as leukemia and ovarian cancer. Clinical trials are currently underway to assess the safety and efficacy of these modified T cells in human patients.
3. Viral Infections
CRISPR's precision extends to viral infections, where it can be utilized to target and cleave viral DNA or RNA within infected cells. Researchers are investigating CRISPR as a potential treatment for diseases like HIV and hepatitis B. By targeting the viral genome, CRISPR could help to eliminate the virus from the host's cells.
In laboratory studies, researchers demonstrated the efficacy of CRISPR in eliminating HIV from infected cells. While still in the experimental stages, these findings hold promise for developing future antiviral therapies.
Ethical Considerations in CRISPR Research
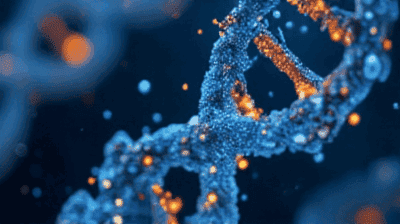
As with any powerful technology, CRISPR gene editing raises ethical concerns that must be carefully considered. Here are some of the key issues:
1. Germline Editing
One of the most controversial aspects of CRISPR technology is germline editing, which involves making changes to the DNA in sperm, eggs, or embryos. Such changes would be passed on to future generations, raising questions about the long-term consequences of altering the human genome. The potential for creating "designer babies" with selected traits presents ethical challenges related to eugenics, social inequality, and unforeseen genetic consequences.
2. Off-Target Effects
While CRISPR is known for its precision, there is still a risk of off-target effects, where unintended parts of the genome are edited. This could lead to unintended consequences, such as the activation of oncogenes (cancer-causing genes) or disruption of critical cellular functions. Ensuring the safety and accuracy of CRISPR applications is essential, particularly in clinical settings.
3. Access and Equity
The availability of CRISPR technology also raises questions of access and equity in healthcare. As gene editing enters the medical mainstream, there is concern that only certain groups may benefit from these advancements, exacerbating existing health disparities. Policymakers need to consider equitable access to CRISPR-based therapies.
The Future of CRISPR Gene Editing
The potential of CRISPR technology is vast, and ongoing research continues to uncover new applications. Here are some directions in which CRISPR is expected to evolve:
1. Agricultural Advancements
CRISPR is also making waves in agriculture, allowing for precise editing of crops to enhance yield, nutritional content, and resistance to pests and diseases. By improving food security and sustainability, CRISPR could play a significant role in meeting the challenges posed by a growing global population.
2. Personalized Medicine
As our understanding of genetics deepens, CRISPR could lead to personalized medicine approaches, where treatments are tailored to an individual's genetic makeup. This could enhance the effectiveness of therapies and minimize adverse effects, particularly for complex diseases such as cancer.
3. Expanding CRISPR Applications
The versatility of CRISPR technology may lead to the development of new CRISPR-based tools and techniques, such as CRISPR interference (CRISPRi) and CRISPR activation (CRISPRa), which allow for more nuanced control of gene expression without altering the DNA sequence itself. These innovations could expand the scope of gene editing and enable researchers to explore new frontiers in understanding gene function.
Conclusion
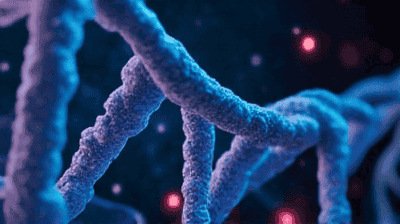
CRISPR gene editing represents a paradigm shift in our ability to manipulate the genetic code, offering unprecedented opportunities to treat diseases that were once deemed untreatable. From correcting genetic disorders to enhancing immune responses against cancer, the applications of CRISPR are both exciting and transformative. However, with this power comes the responsibility to address ethical concerns and ensure equitable access to its benefits.
As the science of CRISPR continues to evolve, so too will our understanding of the potential and limitations of gene editing. It is essential that scientists, ethicists, policymakers, and the public engage in thoughtful discussions to navigate the challenges ahead. By doing so, we can harness the power of CRISPR to improve human health and enhance the quality of life for future generations.